How Does Ketamine Work in the Brain & is it Addictive?
Ketamine's unusual mechanism of action make it reinforcing & psychoactive, but with a low addiction liability.
Ketamine is a hell of a drug. It’s been used for decades as an anesthetic and has recently seen renewed scientific interest for its ability to induce rapid (though temporary) antidepressant effects. It is also used recreationally for its dissociative psychoactivity.
The type of effect you get depends on the dose. Low, sub-psychoactive doses can yield antidepressant effects. At higher doses you get psychoactive effects ranging from alcohol-like intoxication to complete psychological dissociation. At even higher doses, anesthesia.
How can one drug have such a wide range of effects? The answer has to do with ketamine’s mechanisms of action, some which we will explore here in the context of addiction.
One unresolved aspect of ketamine has been its addictive potential. People choose to use ketamine recreationally, so it clearly has rewarding properties which motivate consumption. But is it addictive? How addictive? Given rising popular awareness and the proliferation of ketamine clinics, where people are seeking treatment for depression, is there any cause for concern?
Until recently there has been mixed evidence of ketamine’s addiction liability in the scientific literature. Measuring this liability in animals and determining how ketamine works within the “reward circuitry” of the brain was the focus of a recent study.
(The next two sections provide important background information about how addiction works in the brain. Skip them as needed)
What Is Addiction & How Is It Measured?
There are multiple definitions of addiction. A simple one is the compulsive consumption or seeking of a reward despite negative consequences. This was the definition offered by neuroscientist and addiction expert Dr. Christian Lüscher on M&M #40:
A reward is simply something you like. In the language of experimental psychology, rewards provide positive reinforcement, making it more likely to want to repeat performance of an action or consumption of a substance. If you find something rewarding and you compulsively indulge in it despite negative consequences, you’re addicted.
Different types of rewards have the potential to lead to addiction, not just drugs. Junk food and social media are two obvious examples. People often compulsively indulge in these things despite negative consequences to their physical or psychological health—behavior which can persist with or without much awareness of the detrimental effects.
In experimental settings, scientists assess “reward value” by measuring an animal’s willingness to work for something. Animals will work for food, access to mates, psychoactive drugs, and so on. The more rewarding something is, the more energy you’re willing to expend to get it.
The addictive potential of a substance is not determined solely by its intrinsic properties. It also depends on how easy it is to obtain. When little effort is required to obtain a rewarding drug, the probability of addiction is higher. Make it more expensive to obtain, and addiction is less likely.
Environmental enrichment also matters. Give an animal the option of interacting with novel objects, courting mates, exercising—generally having other stuff to do—and it’s less likely to develop an addiction to an easily available drug or other reward.
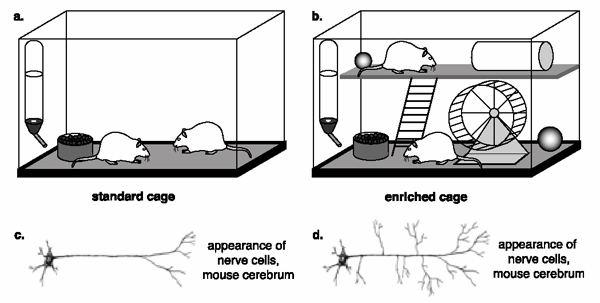
Addictiveness is not an all-or-nothing property. Drugs and other rewards are not either addictive or not addictive. All rewards can be thought of as carrying a certain addiction liability compared to others.
Cocaine is considered a highly addictive drug. This does not mean that most individuals who consume it will become addicted. To the contrary, only ~20% of individuals (either rodents or people) who repeatedly consume cocaine transition to the compulsive drug-seeking diagnostic of addiction. Cocaine is “highly addictive” because more individuals become addicted to it compared to most other drugs.
As Dr. Christian Lüscher told me, “The majority of people can use addictive drugs recreationally without ever losing control.” Why do some individuals show a greater tendency to develop addictive behaviors than others? That’s still a mystery.
Compared to cocaine, drugs such as alcohol and THC have a lower addiction liability. Under conditions where ~20% of animals develop cocaine addiction, a lower percentage develop compulsive seeking to these drugs—lower, but not zero. Drugs like opioids and nicotine carry an even higher addiction liability than cocaine, with addiction rates over 30%.
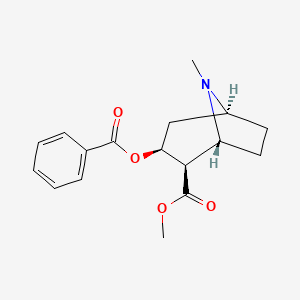
Still other drugs, such as psychedelics, have extremely low addiction liabilities (near 0%). If you give animals easy access to LSD, for example, they are unlikely to self-administer the drug a second time, and virtually none develop addiction. Emerging evidence even suggests they may be able to help treat addiction to other substances.
Why do some drugs carry higher addiction liabilities than others, and what is the addiction liability of ketamine? The answers here require understanding how a drug like ketamine acts within the brain’s “reward circuitry” compared to cocaine.
How Does Addiction Manifest in the Brain?
For casual drug consumption to transform into addictive behavior, a few key steps need to take place in the brain. These changes involve a specific brain network which goes by a few names: the mesolimbic dopamine system, reward pathway, or dopamine motive system. The technical jargon is not so important here. Just know that addictive behavior requires specific changes (plasticity) in certain parts of this system, with the neurotransmitter dopamine playing a critical role.
A crucial node in this system is a connection deep within in the brain. There is a group of dopamine neurons within the ventral tegmental area (VTA), near the top of the brainstem. These dopamine-releasing cells project to the nucleus accumbens, located in a more forward position (closer to the face) near the middle of the brain.
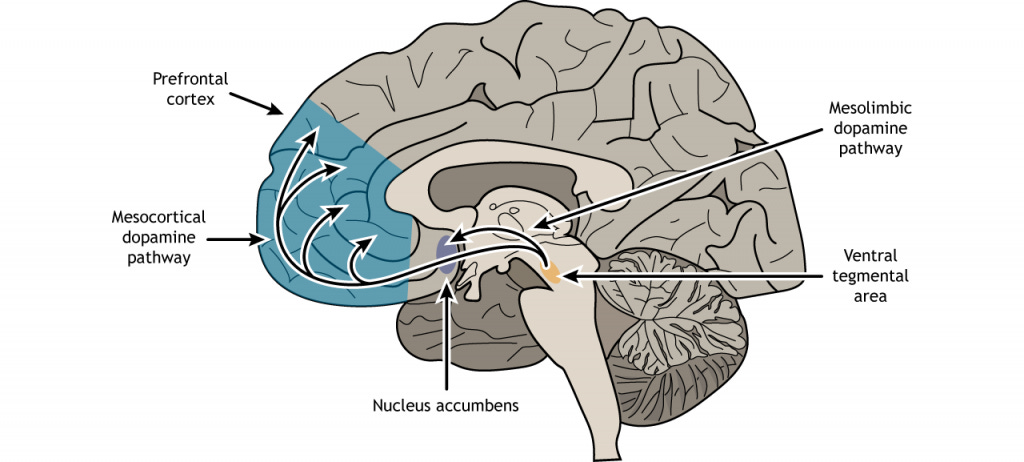
There are dopamine neurons in the VTA and elsewhere that connect to lots of brain regions besides the nucleus accumbens, but the VTA-accumbens connection is one of the most important for reward processing and addiction.
You can spend an entire PhD trying to understand how one piece of one connection in the brain works. We just need the basic gist of this “reward circuit.” Here is a grossly simplified three-step sketch for how the brain transitions to compulsion in addiction:
Step 1: A rewarding drug enters the bloodstream and makes its way to the brain, causing elevated dopamine levels in places like the nucleus accumbens.
“When [addictive] drugs hit the brain, this dopamine is massively enhanced. There’s an increase of dopamine, particularly in the nucleus accumbens but also in the VTA. That is [an] initial trigger that may lead to addiction in some individuals.” —Dr. Christian Lüscher
This dopamine surge is necessary, but not sufficient, to cause addiction. It occurs to some extent when you ingest any rewarding drug or other substance with addictive potential. As a general rule, the more rewarding the substance (the more you “like” it), the bigger the dopamine surge. Non-addictive drugs, such as psychedelics, do not have this effect on dopamine.
For compulsive drug-seeking to develop, further changes must take place.
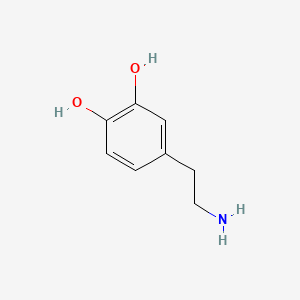
Step 2: Elevated dopamine levels enable synaptic plasticity to happen within the nucleus accumbens.
This region receives input from many brain areas, including the cerebral cortex, which are critical for addiction progression. Elevated dopamine allows the strength of these connections to change—some get stronger, some weaker. In other words, dopamine is a neuromodulator: it influences how neurons within the accumbens “talk” to one another.
As in the first step, Step 2 does not guarantee compulsive drug-seeking will develop. These first two steps—elevated dopamine levels followed by neuroplasticity within the accumbens—are necessary but not sufficient for addiction to take hold.
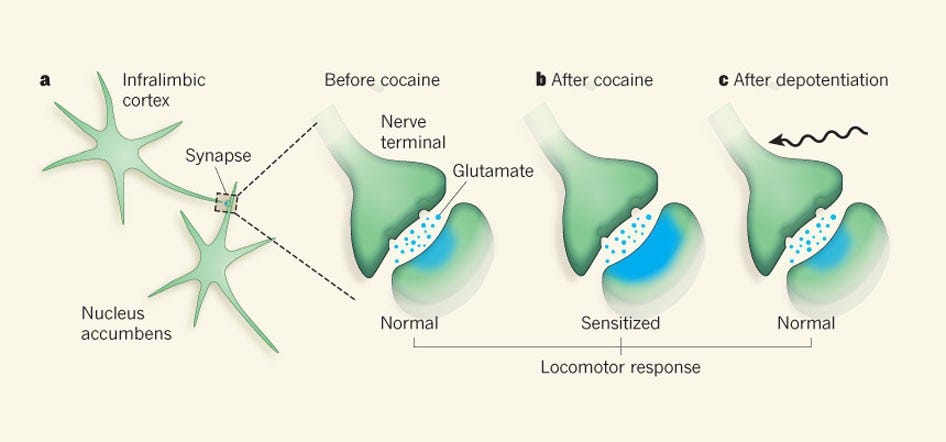
Step 3: The first two steps occur in all animals with consumption of an addictive drug, but only a minority of individuals will transition to compulsive drug seeking. For compulsive behavior to take hold, further changes in structures located more dorsally in the brain (towards the top of the skull) must take place.
So there are three steps: the initial triggering of the dopamine surge, the second is neuroplasticity in the accumbens, and the third then is the relay to the dorsal structures. Only if these three sequences happen, an individual is eventually addicted. —Dr. Christian Lüscher
Again, we don’t yet know why Step 3 occurs only in a minority of individuals, but it does. The details of what, exactly, is changing in the brain during Step 3 are not as well worked out as those of Steps 1-2. This is am area of current research focus.
That’s the basic process by which a drug like cocaine can drive addiction. What about ketamine? Does it drive compulsive behavior in animals? If so, what is its addiction liability compared to other drugs? These questions were addressed in a recent study by Dr. Lüscher’s lab.
Does Ketamine Cause Addiction in Rodents?
A first step to determining whether a drug can cause addiction is to test whether it’s reinforcing. This is assessed by allowing animals to perform a simple action (e.g. a nose poke) to receive a drug infusion. If the drug is rewarding, animals will opt in to receive drug infusions more frequently than a neutral substance (saline). This is exactly what was seen in mice given easy access to either cocaine or ketamine—both drugs were comparably reinforcing.
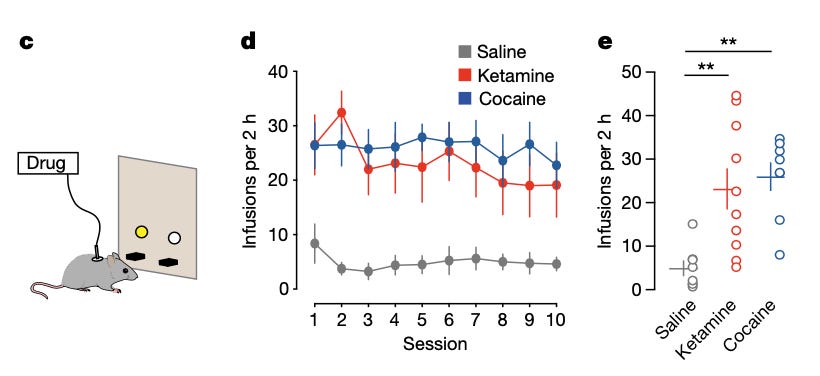
Experiments like this measure whether a drug is reinforcing but not whether compulsive drug-seeking has developed. To measure that, scientists use a behavioral paradigm with a “progressive ratio reinforcement schedule.” First, you train mice to press a lever (i.e. work) to obtain a reward—in this case, either cocaine or ketamine. Then you measure how often they press the lever in a given time period, comparing that to the number of times they press an inactive lever. The more they’re willing to press the drug lever, the more they want the drug.
With progressive ratio reinforcement, you start with a single lever press causing a single drug infusion. After a few sessions, you start requiring two lever presses for each drug infusion. After a few more sessions, three presses are required. You keep doing this until animals reach their “break point,” which is the total number of lever presses they’re willing to make in a session before giving up.
Animals with high break points are engaging in compulsive drug-seeking behavior. On average, when you require a mouse to work twice as hard for its cocaine, it will put in twice as much work in a four-hour session to get the same amount of cocaine it was getting before. When you make mice press a lever three times for every one cocaine infusion, they again work harder to get their fix. A subset of mice do this compulsively, pressing the cocaine lever over 100 or even 200 times in a single session.
With ketamine, you see a different pattern. When required to make one lever press to get one drug infusion, mice press the lever less frequently than they do for cocaine. Over the course of four sessions, they begin pressing the lever more, eventually pressing it as much they would for cocaine. However, when you start requiring more lever presses for each ketamine infusion, their lever pressing behavior decreases—they’re unwilling to work harder to receive ketamine.
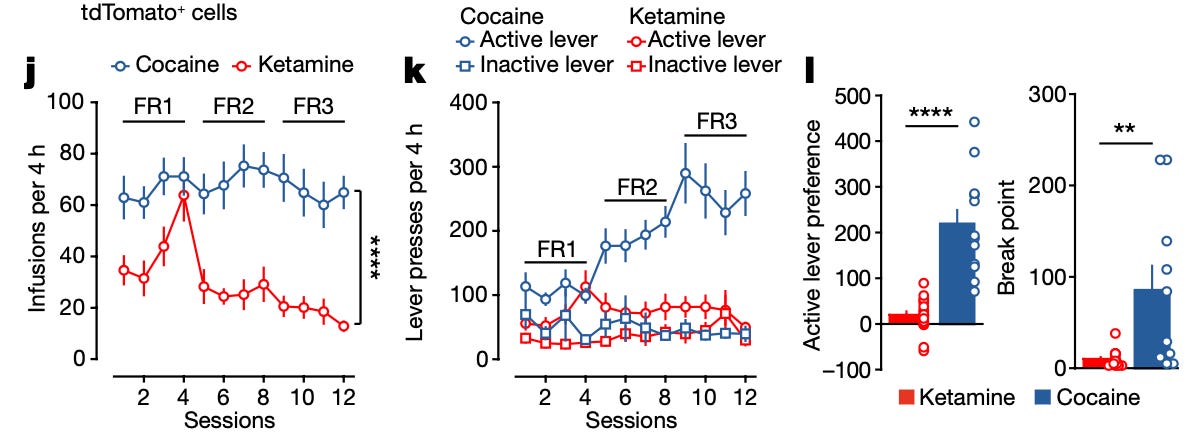
Thus, ketamine shows the behavioral hallmark of a reinforcing drug—mice will choose to self-administer it when given easy access—but it does not lead to the uncontrolled self-administration characteristic of addiction. How can a drug be reinforcing but carry a low addiction liability? The answer lies in how ketamine affects the reward circuitry of the brain compared to cocaine.
How Does Ketamine Affect ‘Reward Circuits’ Within the Brain?
Recall the three-step process for drug addiction outlined above:
Elevated dopamine in key brain areas;
Neuroplasticity in the nucleus accumbens;
Further changes in other regions.
How does ketamine compare to cocaine in terms of Step 1—driving elevated dopamine in the nucleus accumbens? To measure this, scientists used a new technology to measure how much dopamine is being released into synapses and binding to dopamine receptors in the nucleus accumbens. Using a molecular tool called “dLight,” elevated dopamine binds to a dopamine sensor, causing it to emit fluorescent light which can be measured through an optical fiber. More light means more dopamine.
In response to ketamine, the peak dLight response in the nucleus accumbens was nearly as big as that produced by cocaine, but the time course was different. Compared to cocaine, the ketamine response went down faster, meaning that cocaine caused dopamine levels to remain elevated for longer.
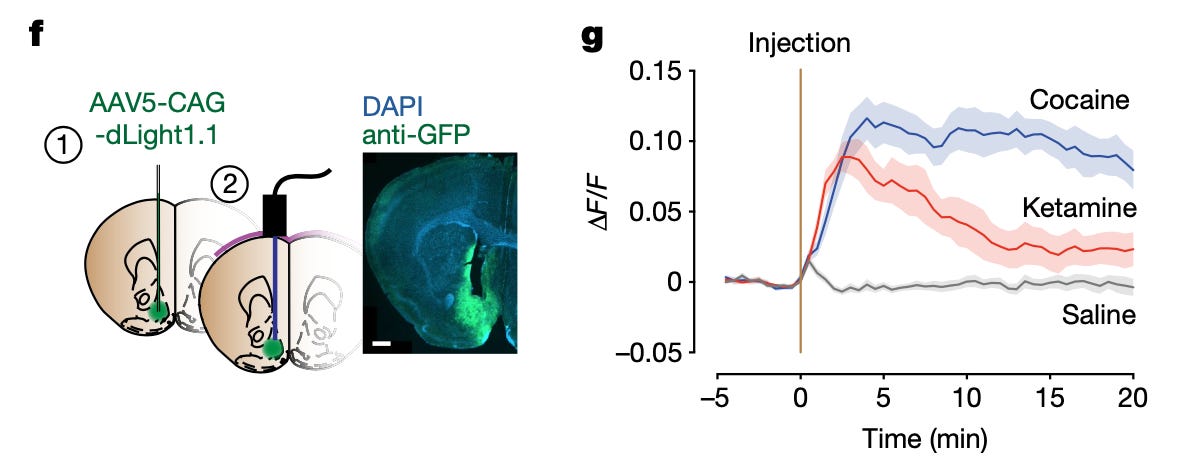
With cocaine, dopamine remains elevated in the nucleus accumbens as long as cocaine remains in the system. In contrast, for ketamine, elevated dopamine levels go down within a few minutes, even while the drug is still in the system.
What about Step 2—neuroplasticity in the nucleus accumbens? It turns out that this was not happening at all in response to ketamine, due to one of ketamine’s major mechanisms of action: blocking the NMDA receptor. This is a special receptor found which is crucial for neuroplasticity. (I have previously written about ketamine’s ability to block this receptor in the context of its dissociative effects.)
Ketamine’s inability to trigger a sustained increase in dopamine, combined with blockade of NMDA receptors, precludes the neuroplasticity required for compulsive drug-seeking behavior to develop. Dr. Lüscher went into more detail in our conversation, but the way ketamine is working within the brain’s reward circuitry seems to be unique.
“To my knowledge this is the first time we had this unique constellation of an increase in dopamine but then not engaging the subsequent cascades of plasticity that we know underpins addiction.” —Dr. Christian Lüscher
What Does This Mean For Humans?
So does this study prove that ketamine is not addictive? No. This study shows that, in mice, ketamine’s addiction liability is much lower than cocaine’s. That does not mean it’s addiction liability is zero. One important thing to keep in mind is that 20 mice were used in the ketamine arm of progressive ratio reinforcement experiments measuring compulsive drug seeking. If they were to repeat this four more times (n=100 mice total), it’s conceivable that, say, a couple mice might develop compulsive drug-seeking for ketamine.
There’s always a question of whether animal studies will translate to humans. Ultimately, you don’t know until you do the necessary human trials. In the case of the brain’s dopamine reward system, we know that the core mechanics of this circuitry are evolutionarily conserved—they work in more or less the same fashion across mammalian species. Even though humans and rodents are different, the same basic three-step process is at play in this network as compulsive behavior develops. That’s why roughly 20% of regular cocaine consumers, whether rodents or humans, eventually transition to compulsion. It’s also why the same drugs that are reinforcing in rodents are reinforcing in humans.
With the rise in use of ketamine as an antidepressant, it was a good idea to study and measure its addiction liability compared to a more well-characterized drug. This work suggests that the addiction liability of ketamine for humans is likely to be much lower than drugs like cocaine. However, it also clearly showed that ketamine does have reinforcing properties—animals will continually self-administer as long as they don’t have to work too hard. That’s something to keep in mind if you contemplate your own potential ketamine consumption.
To learn more about the topics covered in this essay, try these episodes of the Mind & Matter podcast:
Lisa Monteggia: Ketamine, SSRIs, Depression, Psychiatric Disorders & the Brain | #29
Christian Lüscher: Drug Addiction, Cocaine, Nicotine, Caffeine, Amphetamines, Opioids, THC & Neuroscience | #40
Christian Lüscher: How Does Ketamine Work & Is It Addictive? | #90