Dietary Fructose & Metabolic Health: An Evolutionary Perspective
Humans and other apes are more metabolically sensitive to fructose than other mammals. Our bodies are not well-adapted to an artificially fructose-rich environment.
Not medical advice.
Here’s where we are going:
Humans and other apes are more sensitive to the metabolic effects of fructose due to an inherited mutation that may have helped ancient apes avoid starvation.
Fructose has different metabolic effects than glucose and other sugars.
Fructose metabolism in the liver can lead to bad stuff: fatty liver, oxidative stress, inflammation, and insulin resistance.
The modern, fructose-rich food environment is an evolutionary mismatch for our inherited metabolic sensitivity to fructose, which is why fatty liver disease is rising fastest in children.
Arbitrary amounts of fructose cannot be eaten without negative health consequences.
Unless otherwise noted, these papers are the primary references for this post:
Paper 1: Dietary fructose: from uric acid to a metabolic switch in pediatric metabolic dysfunction-associated steatotic liver disease
Paper 2: Nutrition and its role in human evolution
Paper 3: Evolutionary basis for the human diet: consequences for human health
Grapes and raisins are toxic to dogs. Avocados can trigger illness in birds, rabbits, and horses. Squirrels can eat seeds that cause severe poisoning in humans. Every species is “built for” a certain diet. A species' specific metabolic adaptations determine its ability to extract nutrition from foods, sensitivity to food components, and susceptibility to toxins. Different animal species have different metabolic adaptations, which reflect differences in their natural history. Like, duh.
The same holds true within each species, to some extent. For example, human lineages differ in their ability to metabolize fatty acids, starch, and other food components. Substantial metabolic diversity exists among humans, a natural consequence of that fact that humans came to occupy virtually every habitat around the world within the past 100,000 years. In this post, we’ll examine a metabolic quirk that distinguishes humans and apes from other mammals: fructose sensitivity. This metabolic trait arose long before humans walk the Earth, and is shared by all humans alive today.
M&M podcast episodes related to this content:
M&M #185: Sugar & Sweeteners: Are They Really Addictive or Unhealthy? | Nicole Avena
M&M #140: Obesogens, Oxidative Stress, Dietary Sugars & Fats, Statins, Diabetes & the True Causes of Metabolic Dysfunction & Chronic Disease | Robert Lustig
M&M #184: Endocrine Disruptors & Metabolism: Microplastics, BPA, Estrogen, Insulin, Pancreas Biology & Metabolic Dysfunction | Angel Nadal
Primate Phylogenetics: How close are humans, apes, and monkeys?
Humans are apes. Apes are primates. Primates love fruit, right? Most seem to really enjoy it. Fruit is a natural whole food containing not only sugar, which can be used for energy, but vitamin C and other nutrients. Exogenous sources of vitamin C are crucial for primates. Unlike other animals, which endogenously produce vitamin C, we must obtain it from the diet. It’s not surprising that fruit is considered a quintessential health food, and people seem to believe that more is always better. How often do you hear people talk about limiting fruit intake?
This post isn’t really about fruit per se—it’s about fructose, a sugar commonly found in natural fruits and as an additive to processed foods (e.g. high-fructose corn syrup, HFCS). The point of this post is to illustrate how understanding our evolutionary history can provide practical insights into how to think about your own diet.
Fructose is natural, commonly associated with “health foods,” and consumed in the wild by our closest living relatives—from these facts, you might infer that fructose poses little to no risk for your metabolic health. To understand why this is not the case, we need to understand the evolutionary history of our lineage and how it compares to other primates. As discussed elsewhere, tens of thousands of years is more than enough time for significant metabolic adaptions to evolve in a species. It’s therefore risky to make inferences about modern human metabolism based on comparison to our closest living relatives, which we’re separated from by millions of years.
As detailed in this post, early species of archaic Homo began eating more animal and less plant food compared to proto-humans and other apes. Millions of years before any human species ever walked the Earth, the ape lineage evolved a mutation that inactivated a key metabolic enzyme that other mammals possess. Here’s when and where it happened in primate phylogeny:
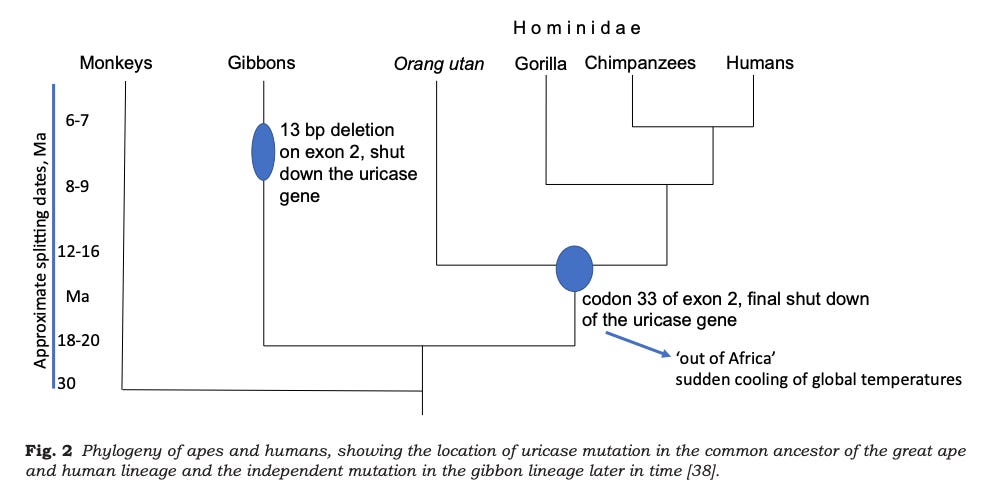
Before exploring the underlying biochemistry and its relationship to fructose metabolism, it will help to understand what drove this mutation. What was the ecological context in which it arose in our prehistoric ape ancestors?
Humans and chimps share a common ancestor that lived around 6 million years ago. All living apes share one that lived about 14 million years ago, and we split from gibbons roughly 18 million years ago. A couple million years after the great ape-gibbon split, 15-16 million years ago, global temperatures rose, prompting the earliest known migration of apes out of Africa and into Eurasia. Later, global climate shifted again, this time in a different direction—temperatures dropped, habitats became more arid, and dry and cold seasons became longer and more extreme away from the equator. There is fossil evidence that Eurasian apes were dying off in this period, likely due to increased starvation. By about 8 million years ago, apes were extinct in Europe and Asia, confined back to Africa.
In was in that ecological context that the gene encoding the uricase enzyme, which breaks down uric acid, was rendered non-functional by mutation. The inactivation actually evolved twice independently: once in the ancestor of all present-day great apes and a distinct mutational event somewhat later, in the gibbon lineage. It’s easy to imagine fruit becoming more sparsely available during this time. In that context, apes with an enhanced ability to store energy from fruit would have been more likely to survive through winters or dry seasons, when food was less plentiful:
The ability of the mutation to enhance the ability of fructose to generate fat creates a survival advantage that would be greater in temperate habitats in Eurasia, where dry/cold seasons would have been greater and longer. Thus, the uricase mutation, by amplifying the effects of fructose to increase fat stores, blood pressure and insulin resistance may have acted as a ‘thrifty gene’, providing survival advantage to fossil apes in seasonal habitats during times of food shortage.
(Source: here)
Whatever the full details of this evolutionary story, the gene encoding uricase accumulated loss-of-function mutations that our ape ancestors inherited. Inactivation of genes is common in evolution, and it’s usually easier to break a gene than to create or resurrect one (a pattern I once studied in the lab).
Let’s get a basic idea of fructose metabolism, how it relates to uric acid, and the metabolic consequences of this mutation for humans living in today’s fructose-rich food environment.
Similar Mind & Matter content:
Article: What Did Humans Evolve to Eat?
Podcast: Diet, Hunting, Culture and Evolution of Paleolithic Humans & Hunter Gatherers | Eugene Morin
A brief guide to fructose metabolism in the liver
Glucose and fructose are simple sugars found in nature, either as free sugar molecules or bound together as sucrose. Despite their structural similarities, glucose and fructose have distinct metabolic consequences in the body.
As far as I’m aware, glucose can be used for energy in every cell of your body and by every organism in known biology. When you ingest glucose, it can be imported into any cell type and used to generate ATP. In contrast, fructose is mostly metabolized in the liver. Fructose metabolites can be used for energy (ATP) production or, if energy is abundant, to stimulate energy storage as fat or glycogen. When the liver’s energy needs are met, such as in a state of overall positive energy balance or with acute overfeeding, fructose metabolites are directed away from ATP production and towards other processes, such as lipogenesis and uric acid formation.
Lipogenesis means fat for energy storage—with enough fat stored in the liver, you have “fatty liver” (not good). We used to think of this as a problem specific to long-term alcoholics. Nowadays, young kids get fatty liver—in fact, it’s becoming disturbingly common. An estimated 5-10% of children in the US have fatty liver today, including over a third of those with obesity. They’re not drinking vodka—they are guzzling high-fructose beverages, such as soda and juice.
The hepatic metabolism of fructose is very different than glucose, as it is insulin-independent, bypasses the process of glycolysis, and increases de novo lipogenesis to a greater extent. Indeed, the hepatic metabolism of fructose is more similar to that of ethanol (Yu et al. 2021).
Source: here
Once you understand that liver metabolism of fructose is akin to that of alcohol, it’s not so surprising that excess fructose consumption leads to liver problems that we used to associate primarily with alcoholism.
Hepatic fructose catabolism (breakdown) is 10x faster than that of glucose, leading to rapid cellular ATP (energy) depletion, uric acid accumulation, and reactive oxygen species (ROS) formation. Fructose generates 100x more ROS than glucose. Uric acid promotes inflammation and oxidative stress in mitochondria. It’s here where humans differ from other mammals: the gene encoding the breakdown enzyme for uric acid is non-functional, resulting in greater uric acid accumulation. Without a uricase enzyme, we have to simply excrete uric acid. Two-thirds of it goes through the kidney, one-third through the GI tract. In other words, when uric acid levels rise, it stimulates inflammation and oxidative stress until your body is able to ship it out to your toilet.
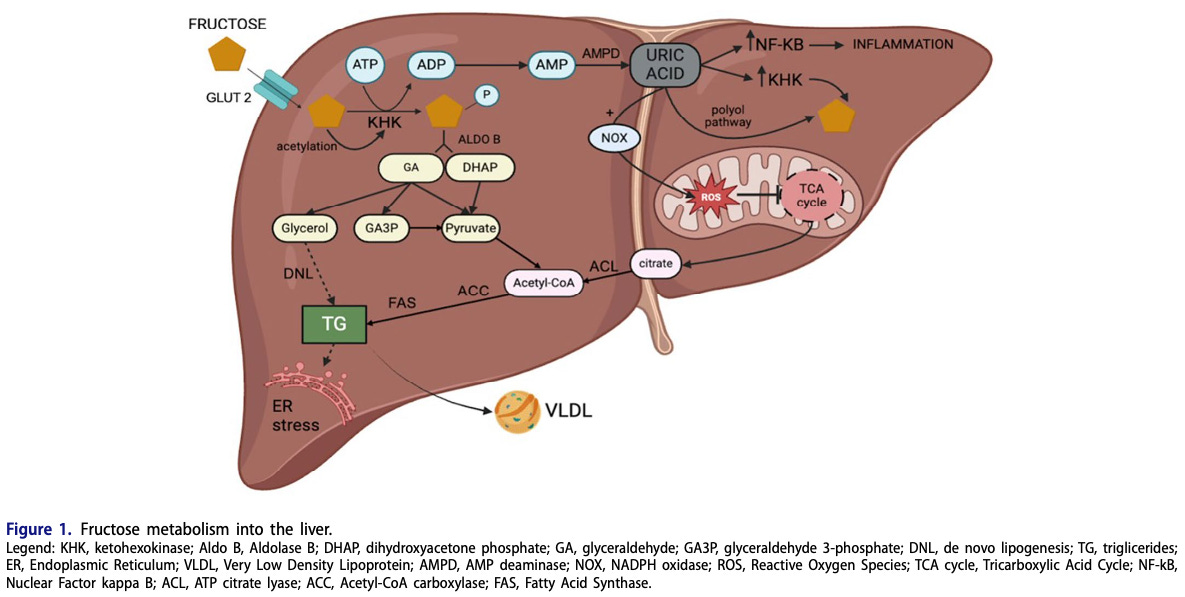
Humans have the highest uric acid levels of any mammal. This is probably because we not only lack a functional uricase enzyme like other apes, but our food supply contains ample amounts of fructose, often in concentrated liquid form. Without fiber and other physical food mass to slow down absorption, every can of Dr. Pepper amounts to a large liquid bolus of fructose going straight to your liver to elevate uric acid levels.
Without going into much biochemical detail, excess fructose consumption by humans is thought to lead to insulin resistance, fatty liver disease, and related issues via de novo lipogenesis and uric acid accumulation. Animals without the ability to quickly break down uric acid are naturally more prone to these outcomes if they’re consuming sufficient amounts of fructose. Recall what we observed about fructose consumption in present-day humans: children are consuming roughly the same amount of fructose (as percent of calories) as wild frugivorous apes, except that they’re leading sedentary lives and obtaining their fructose from sugar-sweetened beverages and other ultra-processed foods. Again, rates of fatty liver disease are rising fastest among young children, a condition we used to see only in middle-aged alcoholics. Fructose consumption is too high, and eating an apple a day on top of this won’t keep the doctor away.
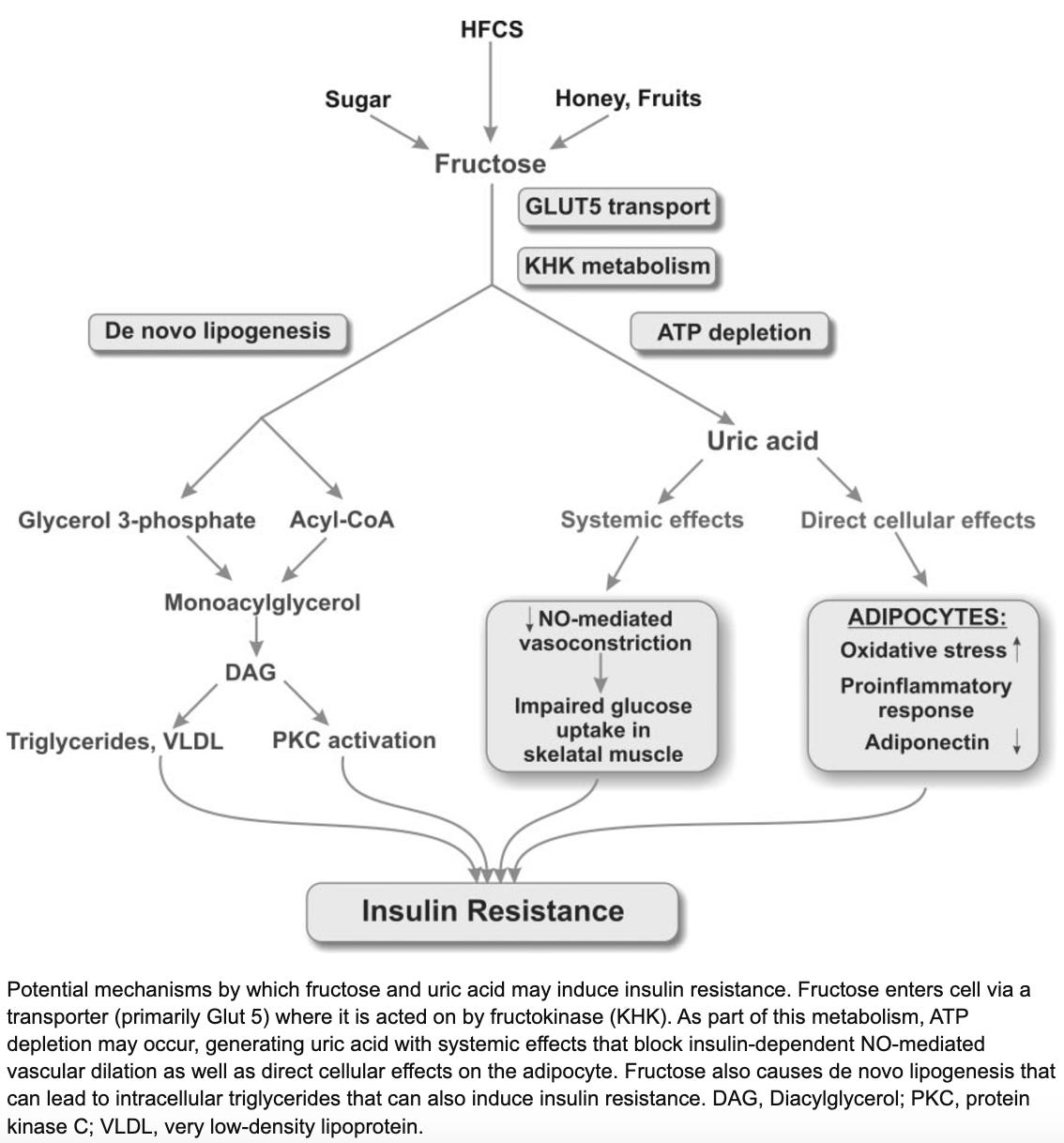
Fructose consumption & uric acid levels in humans vs. other mammals
If humans and other great apes share the same non-functional uricase gene, shouldn’t they also be prone to uric acid-dependent metabolic dysfunction from excess fructose consumption? I mean, don’t chimps eat a lot of fruit? If the above logic holds true, shouldn’t chimps suffer from insulin resistance and fatty liver disease at least as much as modern humans?
Wild chimps eat a diet consisting of leaves and other plant material, fruit, insects, and small animals (when they can catch them). It’s estimated that ~50-60% of their diet comes from fruit, in terms of the number of grams consumed. In terms of the percent of calories from fructose, this is estimated to be in the ~10-20% range. As it turns out, this is comparable to estimates of fructose intake for modern children, which are in the 15-18% range (possibly even higher today, particularly in unhealthy sub-populations that consume the highest levels of ultra-processed foods).
So, roughly speaking, modern human children and wild chimpanzees are eating comparable amounts of fructose in terms of calorie percentage. Now consider other obvious lifestyle and consumption differences between the two groups: chimpanzees roam around all day, foraging and climbing. They get their fructose from wild fruit, which contains fiber and other nutrients. Even if you matched fructose levels gram for gram, that mode consumption will yield very different metabolic effects than sitting around drinking Dr. Pepper or Sunny D.
Modern human children lead a much more sedentary lifestyle, with a high percentage of their fructose coming from ultra-processed sources like juice boxes, sodas, and foods with high-fructose corn syrup added to an already calorie-dense (and often fiber-free) products. Just think about how much excess fructose will come from drinking multiple sodas or juice boxes per day (common among children), especially for sedentary people. Multiple doses of liquid HFCS, devoid of fiber, straight into livers with full glycogen stores. This is the perfect recipe for fatty liver and uric acid formation—no wonder children’s livers are looking more and more like those of career alcoholics.
As with most things in biology, balance is important. For example, there is also a downside risk to having too low uric acid levels. That being said, the fructose-rich food environment we live in, together with the lack of a functional uricase enzyme, means that people are much more likely to suffer the consequences of too much uric acid rather than too little.
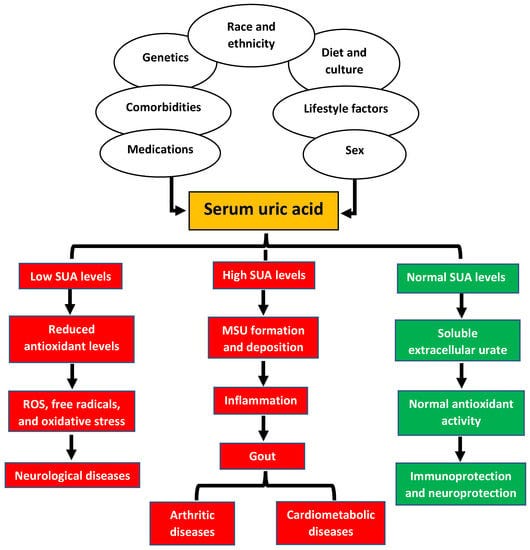
How much fructose is in modern food anyway?
Fructose content in fruits & industrial foods
Recall the basic types of carbohydrates. For our purposes here, we want to focus on simple sugars that contain fructose—either fructose itself or sucrose (table sugar), which is composed of single fructose and glucose molecules bonded together.
The total fructose content of any sugar-containing food will be its fructose content plus one-half of its sucrose content. The sugar content of most fruits is some combination of glucose and fructose, either as free simple sugars or bound together as sucrose. The exact proportion varies between types of fruit and will naturally vary to some extent between harvests and producers. Here’s a basic comparison of the average sugar content of various fruits:
Notice some of the basic patterns:
Most fruits contain a combination of glucose and fructose, which may or may not include much sucrose.
The fruits with the lowest overall fructose content also have lower absolute sugar levels: tomato, lime, lemon, avocado, and casaba melon.
Most fruits with the highest overall sugar content also have high levels of either free fructose or sucrose (or both).
The sweetest-tasting fruits are often the ones with high overall sugar content, which usually includes a high proportion of fructose.
Consider fructose levels in common whole fruits compared to a can of Coca-Cola. A single Coke can have about 40 grams of High-Fructose Corn Syrup (HFCS), which is 20 grams of fructose. Taking into account the average sugar content of grapes, you would have to consume roughly 50 grapes to get 20 grams of fructose. The exact number would depend on the specific type of grape, but when was the last time you ate 50 grapes in one sitting? Now think about how easy it is to drink one can of Coca-Cola and how common it is for modern people to drink multiple per day.
I’ve often observed people eat a few grapes or order a side of fruit with meals and say something like, “I should be at least a little healthy [lol]!” Much of the time, those people are just adding additional sugar to an already sugar-rich diet, including fructose. They’re supplementing their diet of HFCS-laced food and beverages with more sugar from whole fruits. This will lead to a very different metabolic outcome compared to someone who replaced all processed foods with added sugar with whole fruit—again, this would be true even if the fructose content was matched, gram for gram.
Or consider the huge volumes of fruit juice people routinely give to children, often under the guise that it’s “healthy” because it contains vitamin C or whatever. No wonder rates of childhood fatty liver disease have been skyrocketing.
Here’s a simple graphic I found online that gives the fructose content of common processed foods:
And here is a study that measured the sugar content of common ultra-processed beverages:
Evolutionary mismatch: Fructose-sensitive apes in a high-fructose world
15-20 million years ago, the uricase gene acquired a progressive number of mutations that eventually silenced the gene completely. As stated above, this occurred in the context of climactic change that was making things inhospitably cold and arid in the Eurasian habitats apes had made it into. The fossil record indicates high levels of ape extinctions in those habitats. It’s easy to imagine how uricase inactivation would have provided a survival advantage: apes that were more metabolically sensitive to fructose would have more easily been able to transform dwindling natural fructose supplies into stored energy (fat or glycogen). Humans and other living apes are descendants of the ape lineage that survived that prehistoric period, who passed on the inactivated uricase gene to us.
Fast-forward millions of years to the modern human condition: We have effectively unlimited access to calorie-dense foods, including hyper-palatable processed foods with added sugars. Fructose is abundant, often in forms that allow for fast absorption of concentrated doses compared to natural sources like whole fruits. Although our living ape cousins also lack uricase, they lead non-sedentary lifestyles in the wild, where the rate of fructose absorption is limited by it’s presence in fiber-containing whole fruits.
As with pharmaceutical or street drugs, the concentration and form factor influence the effects of a substance, including its reinforcing potential. Consuming powdered cocaine (insufflated) has different effects from chewing coca leaves. The raw leaves still contain stimulant molecules, but the process by which powder cocaine is produced results in a more potent and addictive substance because the stimulant is more highly concentrated and hits the bloodstream faster based on how it’s consumed.
Even if two people consumed the exact same amounts of fructose, one by eating whole fruit and the other by sipping store-bought beverages, the metabolic effects would not be the same. It takes more time to chew and swallow grapes than to drink liquid HFCS. The fiber and physical material the grape-derived sugars are embedded in slows absorption rate. The sugar-sweetened beverage provides a faster and stronger hit than the fruit does, which will tend to make it more reinforcing and easier to consume in excess, leading to more of the de novo lipogenesis and uric acid formation discussed above.
Here’s how fructose content in the US food supply trended from 1970-2004:
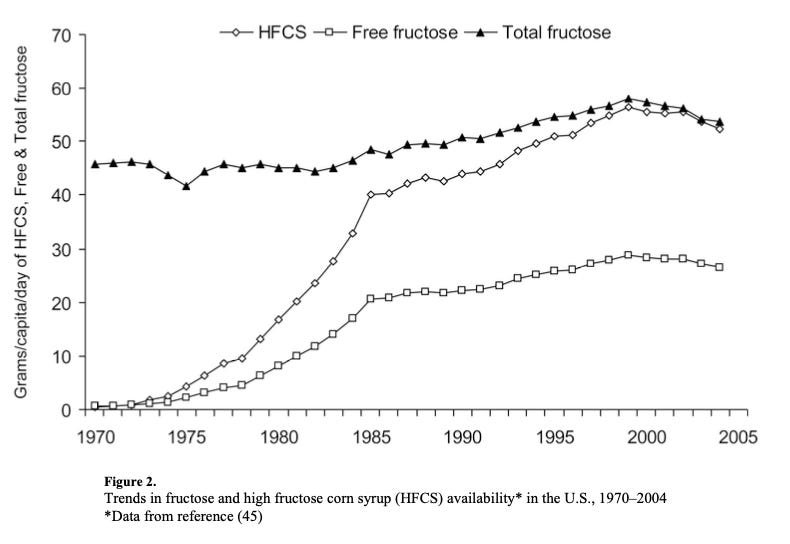
As I’ve pointed out before, these graphs of supply do not correspond to intake per se (the two variables correlated to some unknown degree). As you can see, fructose supply increased across much of this interval, especially high-fructose corn syrup (HFCS). Things tapered off a bit from 2000 onward. Some people point to that as evidence that sugar has little or nothing to do with obesity and metabolic dysfunction, which has continued climbing since 2000. That’s a weak argument—the drop in supply since 2000 was much more modest than the dramatic increase over previous decades, which means that absolute levels of fructose are high, and still inevitably occurring within a context of positive energy balance (caloric surplus) for most people. These are perfect conditions for fructose to stimulate lipogenesis and uric acid formation, in a species that inherited fructose sensitivity from ancestors that likely survived periods of mass starvation. Moreover, there is evidence that calorie intake from specific sugar-sweetened categories, like soda, continued to climb over time.
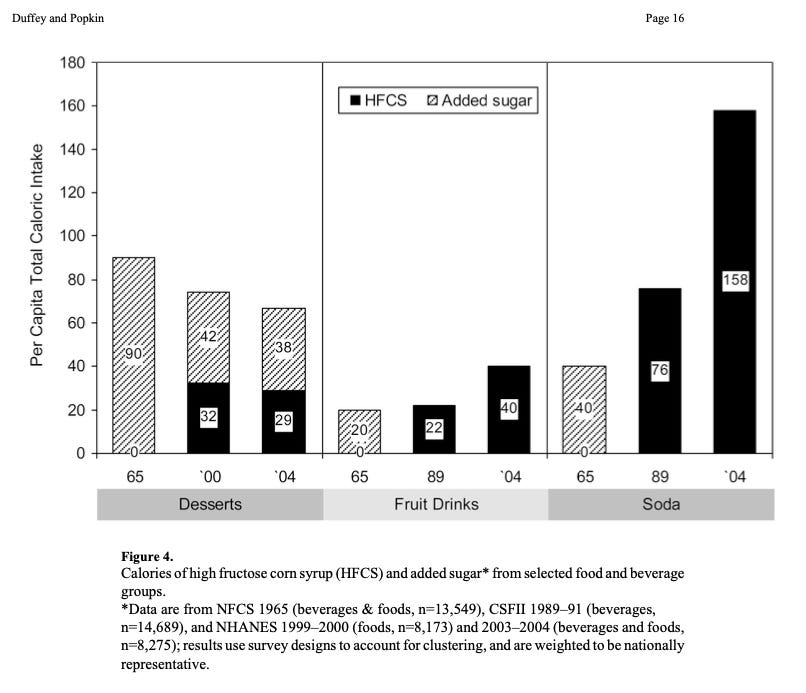
Not only do we have a natural fructose sensitivity due to our enzymatic inheritance, but the modern environment has exposed us to increasing amounts of metabolism disruptors, generation by generation. For examples, microplastics like BPA lead to an exaggerated insulin response. Because each generation of people born since industrialization has been exposed to higher and higher levels of such metabolism disruptors, present-day humans are even more prone to things like insulin sensitivity than previous generations. Even with a tapering off of fructose and caloric sweetener content since 2000, young people today are exposed to more fructose-containing foods, artificially sweetened beverages, and metabolism-disrupting chemicals than ever before—starting at early ages, including while still in the womb.
On top of the inherent fructose sensitivity humans have compared to other mammals, we’ve created a environment swimming with high levels of concentrated, easily absorbable fructose and metabolic disruptors that mess with core aspects of metabolism, like insulin signaling.
All of these things point in the same general direction: metabolic dysfunction, including fatty liver disease, insulin resistance, and obesity.
Learn more about dietary sugar, fructose, and metabolism disruptors:
M&M #184: Endocrine Disruptors & Metabolism: Microplastics, BPA, Estrogen, Insulin, Pancreas Biology & Metabolic Dysfunction | Angel Nadal
M&M #145: Epigenetics, Hormones, Endocrine Disruptors, Microplastics, Xenoestrogens, Obesogens & Obesity, Inheritance of Acquired Characteristics | Bruce Blumberg
What is fructose good for?
When I ask a Large Language Model like Grok of ChatGPT if fructose is good for you, the results are telling. The “pro-fructose” results tend to be things like: it is a source of energy, has a low glycemic index, and is sweet and found in fruits.
Is fructose a source of energy? Well, yes, but there are many dietary sources of energy, and this is hardly an argument that fructose has some kind of specific energetic benefit. While it’s true that fructose has a low glycemic index, this also isn’t a unique property of fructose or an argument that fructose is specifically beneficial in this regard. It’s certainly true that fructose is sweet. This is a major why food companies use fructose and HFCS formulations—it makes processed food more palatable and therefore more reinforcing, facilitating sales. We may subjectively like sweetness, but that’s not an argument that fructose is good for health. And while fructose is indeed found in natural fruits, that is once again not an argument for the benefits of fructose per se.
I think it’s fair to think of fructose as a dose-dependent liver toxin, not unlike ethanol. Plenty of things are toxic in a dose-dependent fashion. Below a certain threshold, it’s not a big deal (or even beneficial). Above that threshold, you get problems—in this case, things like inflammation and oxidative stress. The "toxic dose level" is a function of your consumption pattern (the literal dosing schedule) and other contextual factors, such as the energy demands imposed by your behavior (how active you are) and other components of the diet that affect metabolism (e.g. whether the soda cans you drink from are laced with BPA).
If you were to somehow cut all fructose out of your diet, it’s not clear to me you would have a problem, as long as that wasn’t causing you to lose out on important nutrients. For example, citrus fruit is the biggest source of vitamin C for many people. If cutting out all fructose intake meant cutting out all of your vitamin C intake, that would be bad. But would your health be negatively affected if you cut out all fructose without doing this? It’s not clear to me that you actually need fructose in any way. Some intake is probably not harmful, such as the modest amounts that come naturally with a moderate intake of fruits that contain key vitamins and other nutrients. (If there is some crucial beneficial effect that’s unique to fructose, please let me know in the comments).
Similar written Mind & Matter content:
Sugar Has No Impact On Obesity: Just-so stories in metabolic health
Should you eat fructose?
I’m not in the business of giving health advice (none of my content is medical advice), so I’ll keep this short and sweet (ha!). Here’s what my personal approach to fructose consumption would be, depending on whether I was metabolically healthy or unhealthy.
I’m currently metabolically healthy—good insulin sensitivity, no signs of fatty liver, lean and muscular, etc. I avoid processed foods and added sugars as much as I reasonably can—vigilant, but not fanatical. I also eat fruit, sometimes. It’s a side dish or snack here and there. There’s nothing wrong with consuming some fruit, especially given my active and otherwise healthy lifestyle. But should I be eating as much fruit or fructose as a chimpanzee? I don’t think so, especially since we know that archaic Homo and Homo sapiens evolved away from the plant-heavy diet of other apes, and humans have higher serum levels of uric acid than even our closest living ape relatives.
If I were metabolically unhealthy, I’d aim to cut out fructose completely. “Metabolically unhealthy” here means any combination of the things associated with metabolic syndrome: obesity, insulin insensitivity, fatty liver, etc. This would mean not only cutting out obvious culprits like sugar-sweetened beverages and processed foods but even fructose from natural, whole foods like fruit. Yes, that’s right: if I had fatty liver or diabetes (or even prediabetes), I would stop eating fruit. It’s easy enough to supplement with liposomal vitamin C. The simple idea here is, with fatty liver or diabetes (the two are highly correlated), you want to stress your liver as little as possible. This was something I discussed with Dr. Robert Lustig on M&M #140:
NJ: Let’s say you’re talking about someone with type II diabetes [or fatty liver disease] whose goal should be to reverse that. My inclination would be to cut out fructose completely, until [you’re metabolically healthy].
RL: I agree with that. If you have type II diabetes, almost assuredly you also have fatty liver disease. The correlation between fatty liver disease and type II diabetes is extremely tight. It’s almost for sure that the reason for your diabetes is because your liver is not working right, and because your liver’s not working right your pancreas had to make extra insulin to make your liver work right, and now your pancreas is burned out. And so you’ve got to give your pancreas a chance to work properly, and the only way to do that is to make your liver work properly.
You’ve got to burn off that liver fat. You’ve to get rid of that liver fat in order to fix the problem. A ketogenic diet will do that… if you have type II diabetes, and you have fatty liver disease, the best thing to do is give your liver a rest. And the best way to give your liver a rest is don’t challenge it—fructose is a primary challenge. If you have type II diabetes already, best not to eat fruit, until the diabetes is resolved. And then you probably add it back in, in reasonable amounts.
Of course, fructose is not the only thing that poses a challenge for the liver—ethanol and pharmaceuticals are challenges, too. How much of a break you give your liver week-by-week is up to you. The hyper-modern environment presents us with many challenges that our bodies are not well-adapted for. Compared to other mammals, heightened sensitivity to the metabolic effects of fructose is something all living humans share. People’s livers are more stressed than ever. Mountain Dew, Minute Maid, and HFCS probably have more to do with this trend than alcohol: