What is Psychological Identity? | Part IV: The Neuroscience of Dissociative States
The neuroscience of ketamine-induced dissociative states & neural basis of non-ordinary states of consciousness like dreaming, mindful awareness, and flow states.
This is Part IV in a multi-part essay exploring the biological underpinnings of psychological identity. Links to the other essays in the series, and the podcast episodes that informed them, can be found at the bottom of this post.
Dissociative Phenomena: A Reprise
In Part I, we saw that a certain drugs possess the ability to induce dissociation—states of consciousness where some of the mind’s ordinary associations, including your own self-perception, are temporarily suspended or diminished. Dissociative drugs do this by blocking molecular coincidence detectors (NMDA receptors) on neurons, messing with your brain’s ability to integrate signals of co-occurence. You will have some trouble remembering the details of these episodes, describing them with words like “strange” or “loopy” and likening them to dreams or out-of-body experiences.
In Part II, we explored how dissociations can be induced by changing the pattern of sensory inputs the brain receives from the outside the world. They can be deliberately engineered, as with the specific perceptual dissociations of the rubber arm illusion, or occur naturally, as with dreaming.
With the rubber arm illusion, stroking a rubber hand in close spatiotemporal alignment to your real one causes you to associate the inanimate object with your self-image (ego). You concurrently dissociate from your actual arm, failing to perceive physical touch sensations applied to it. This happens even with full knowledge of the trick. Your subjective experience is dictated by patterns of multisensory integration, not your knowledge of the objective state of the world.
When you enter the vivid, hallucinatory, dissociative dream states of REM sleep, something related occurs. Instead of cleverly hacking sensory perception to dissociate a single body part from your self-image, all external inputs to the brain are attenuated—eyes are closed, body motionless, etc. With physical sensation dialed down, your brain conjures dream images, unfolding with a logic and rhythm distinct from alert, waking consciousness. Dreams also typically involve a dream avatar in place of your everyday ego. The avatar is not the you reading this, as it is dissociated from the memories, sensations, and executive decision making associated with your default waking experience.
Something about your brain’s ability to integrate multisensory information—the way that multiple channels of information flow into the brain and correlate with one another—seems to be critical for how it tags specific elements of it’s sensorium as “me.” Unlike the static, molecular motifs we explored in Part III, which allow your immune system to distinguish self from non-self at the level of individual cells, the brain makes this kind of distinction by “reading” dynamic patterns of electrochemical activity across populations of neurons.
Neuroscience is not yet at the point where we have a precise, mechanistic accounting of this stuff in terms of all the specific circuits and computational details involved. Even if it was, we would still need a way to understand and talk about this stuff in psychological terms. (Intrepid readers interested in how neuroscientists think about this stuff in mathy detail are referred to work on attractor dynamics).
Can we come to a simple, intuitive explanation for what dissociative states are as psychological phenomenon, rooted in the biology? To attempt this, let’s explore some of the general neurological phenomena related to how your brain focuses attention on and disengages specific portions of its information menu. With this understanding, we will have a neuroscience-based framework for thinking about other psychological experiences, such as mindful awareness and flow states. This will help us understand dissociation by contrast and make sense of the latest results on the neuroscience of ketamine-induced dissociative states.
Top-down & Bottom-Up: Information Flow in the Brain
You can think of the nervous system as a series of processing layers. The “bottom” layer is composed of neurons in direct contact with the world outside the brain. These neurons are sensors specialized to detect certain types of physical stimuli. Examples include the sensory neurons in your retina, which detect photons of light, and those of your skin, which detect mechanical stimulation. These sensors are the “bottom” of the nervous system, sending information “up” into the brain. Your primary sensory cortices—visual cortex (sight), somatosensory cortex (touch), and so on—can be thought of as “middle stack.” Higher-order association areas, which integrate inputs across more specialized sensory areas, are the top of the stack.
With each layer of processing, the sensory information flowing forward from the bottom (“feedforward”) gets transformed. Different bits can be enhanced, compressed, filtered. Illusory sensations can even be spliced in based on expectations stored in memory. Recall again the rubber arm illusion: phantom sensations of touch associated with the fake arm were spliced in while your visual attention was fixed on it; sensations of physical touch to your actual arm were filtered out while it has hidden from view.
As you ascend the processing hierarchy, neurons closer to the “top” send projections down the neural stack (“feedback”), carrying information about memories, expectations, etc. Feedback connections emanating from deep within your brain edit bottom-up sensory information, determining whether and how it will be integrated with information already risiding within. A lot of this happens before perceptions are rendered. Much of the work done by top-down, feedback circuits occurs via neuromodulators—chemical messangers like serotonin, norepinephrine, or acetylcholine—which modulate how neurons respond to the signals they receive.
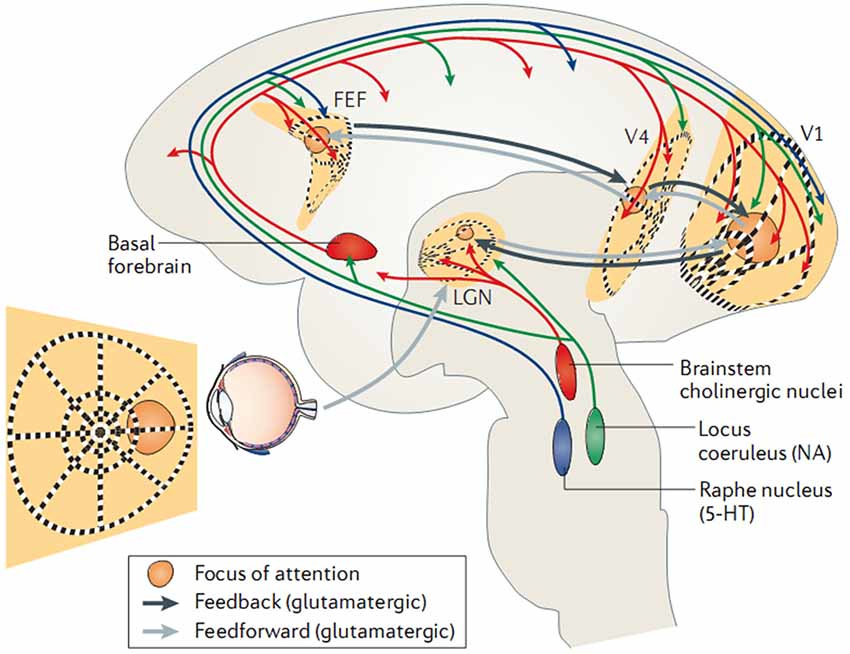
As you read this, your eyes move left-to-right. Imagine instead your eyes were still but a motorized screen moved to your left, creating the exact same shift in visual input. How does your brain tell the difference? In the first case, a motor command is sent to your eye muscles (“move eyes left-to-right”). Coming from your motor system, this pattern mixes together with one coming from your retina. Like ripples emanating from two stones tossed into different ends of a pond, a new pattern is created where they collide.
Interference patterns, created by mixtures of sensory and motor information, are distinct from the “pure” sensory ripples generated if your eyes are still and the world shifts around you. Networks in the brain can read out the difference between these patterns—one caused by you and the other by not you. By reading these interference patterns, your brain can classify whether a given change in sensation was caused by the movement of your sense organs or the independent motion of an external object. This type of integrative work, together with other aspects of cognition, is often done by patches of cerebral cortex called “association area” which receive multisensory inputs from across the brain.
Have you ever gazed out the window of a train car as it slowly begins moving, while looking at an adjacent, stationary train? For a moment or two, your experience is that the other one is moving. The shift in visual input combined with steady pattern of muscle contraction to hold your head and eyes still tricks the brain into concluding, “external object is moving.” This misperception is quickly resolved by moving your head, creating a distinct sensorimotor interference pattern the brain interprets as, “my body is moving.”
Different phenomenon, similar idea: tickling. If somebody else applies light, erratic touch to your stomach, you giggle and squirm. When you apply the same stimulation, you don’t. Coming from someone else, the sensations are surprising—you can’t predict exactly what comes next—because your brain does not have access to the motor commands driving the other person’s fingers. When you try to tickle yourself, your brain does have access to these commands. Your motor cortex actually sends a copy of them to sensory regions. An efference copy, moving in the top-down direction, tells your somatosensory system what pattern of bottom-up touch sensation to expect. The salient, pleasureable-but-annoying-please-make-it-stop! feeling never arises because the element of surprise is filtered out
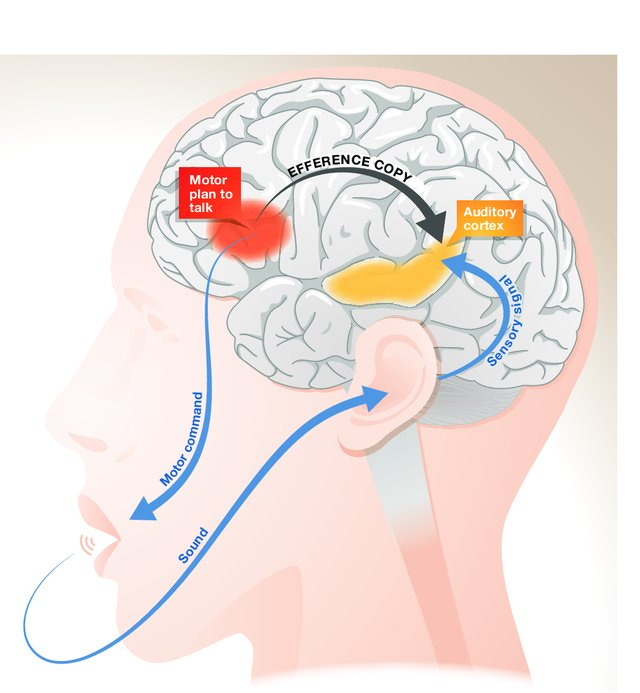
Exactly how sensory and motors signals mix together in the brain—how they correlate and interact with one another—is important for determining the character of your experience. Two identical patterns of sensory input coming into your brain can result in distinct interference patterns emerging “higher up” depending on the neural context each flows into. Toss a stone into a placid, glassy pond and you get one pattern. Toss the same stone into a pond already choppy with waves, you get another. This is akin to the difference between whether or not your experience elicits uncontrollable laughter, or if it’s imbued with a sense of you-ness.
Related factors influencing how this you-ness shows up are the levels of attentional focus and motor fluency you exhibit moment-to-moment, which further set the neural context—the pattern of choppiness in the pond.
Attention, Mindfulness & Flow States
When you pay attention to something, it’s sensory attributes are in crisp focus and you’re prepared for appropriate engagement. When attention lapses, you stop seeing them as clearly and can be caught off-guard by a change. Perhaps you start daydreaming—attention disengages from the sensory information flowing into the brain. Your subjective experience becomes dissociated from the visual data entering through your open eyes. Consciousness becomes populated by imaginations bubbling up from within. Top-down information streams in your brain are “beating” bottom-up sensory streams in a kind of neural tug-of war until a new, extra salient external stimulus snaps your attention back outward.
Attention and cognition are scarce resources. As with any organ housed within a living, mortal organism, the brain must economize how its resources are deployed. Organ systems must be energy efficient in order to ensure survival and reproduction (the ultimate evolutionary imperative). Brains are metabolically expensive, using lots of energy even when at rest. This is especially true of clever primates with disproportionately large brains for their body. Chess players can burn up to 6,000 calories per day during tournaments, just sitting and thinking.
Attention is selection mechanism for determining which bits of the brain’s swirling information cocktail get subjected to more energy intensive forms of neural processing. Which elements of our potential experience actually make it into conscious awareness—which information streams win the neural tug-of-war—is a function of how big a splash they make in your mental pond (salience). This is why something like a loud, abrupt clap is often needed to snap someone out of a daydream.
Even when you’re alert and aware of your surroundings, attention can become hyper-focused on a particular subset of what’s in front of you. The “spotlight of attention” can focus so intensely on one element of a visual scene that your brain filters out other elements of possible awareness, even though information about them enters via the retina. The salience of objects we attend to is heightened, others diminished.
A famous exercise in selective attention—follow the instructions carefully, in full-screen mode (no cheating!):
The brain adjusts the salience of neural representations by changing the gain of the neurons representing the objects of attention. In the basketball example above, focusing on the ball and the white shirts dialed up the gain on neurons representing those objects. The ball is basically represented by various patches of neurons in your visual cortex firing in a particular pattern. With attention on the ball, those neurons fire more or less in the same pattern, but do so more vigorously, largely because feedback circuitry changes the neurochemical environment bathing those cells. With attention, they are “speaking” more loudly, making a bigger splash in your mental pond and therefore having a larger influence on the overall pattern of choppiness dancing throughout it.
Attention can become so focused on the present task that you “lose yourself.” The you-ness permeating default waking experience fades away. Mindfulness meditation is a practice that trains attention such that you get better at noticing when you start thinking about your perceptions. Thinking is essentially a set of cognitive processes taking place in between sensation and action. In a flow state, you become so engrossed in what you’re doing that there’s no neural space left between sensation and action. A seamless experience of doing and perceiving, minus the narration, unfolds. Ancient peoples would have called it a trance.
Flow states are most commonly experienced when your current level of behavioral fluency is matched the level of competence demanded by present circumstances. This is especially likely when it’s a more difficult, newly acquired competency. Executing a series of skilled actions for the first time is exhilarating. You may know the feeling from times of exceptional athletic performance, artisanal craftsmanship, and so forth.
Total sensory absorption, together with the lack of thinking needed to fluidly execute well-learned behaviors, means there is no need for thoughts to intervene between sensation and action. While learning a new skill, you have to stop and think about it. Once fluent, you just go with the flow.
Flow is not limited to overtly physical skills. Computers coders, writers, and intellectuals scribbling on white boards achieve it also. The flow states emerging from the right balance of attention, fluency, and challenge feel the way they do because they’re tied to brain states—overall patterns of choppiness—distinct from those of default consciousness.
Different brain states are determined in large part by the brain’s neurochemical environment—the specific composition of neuromodulators (serotonin, dopamine, etc.) in your mental pond. These chemicals modulate how neural circuits respond to inputs and therefore the types of interference patterns arising in the present sensorimotor context. In highly simplified terms, a flow state may be accompanied by higher-than-normal levels of a neuromodulator like norepinephrine, which makes neural representations of the objects of your attention “louder,” increasing their influence in the neural tug-of-war.
When the stakes are high (physiological arousal) and you know what you’re doing (fluency), as in championship sports, flow states are primed to arise. MJ probably didn’t deliberate about his famous “last shot.” He was in a trance:
Metacognition, Memory & Dissociation
Because thinking is attenuated in flow states, there’s also less thinking about thinking—metacognition. With the narrative voice of the ego boxed out by the seamless, reciprocal exchange of sensation and action, there’s no room for a narrator to spin a story about the experience. Without a story formulated, there’s no story to remember. The experience is just a happening—sequences of sensorimotor percepts coming and going.
Diminution of metacognition is characteristic of both flow and dreams, probably related to the low-fidelity memory encoding of these states. When we remember dreams at all, our memories are fleeting and fuzzy, rarely involving clear instances of “executive cognition.” Consider how much of your time you spend reading text, like this, on a screen. Have you ever had a dream where you’re reading anything, let alone cogitating on it? I haven’t. (At least not that I can remember).
The link between metacognition and memory is likely also related to why people who were just “in the zone” during high stakes events have trouble recalling details. People who go through high stakes experiences often fail to encode salient aspects of the experience in memory. This is why eyewitness testimony is not always reliable.
Victims of trauma may relate. They often report slipping into dream-like, out-of-body experiences while the trauma took place. In such non-ordinary states, “higher” brain networks are not crafting a story about what’s going on, at least not in the way they normally do. Because information is being encoded in an unusual manner, perception and memory formation do not proceed as usual. There was a happening, but much like a dream or dissociative drug experience, the details are hazy.
“Walk us through what took place.”
“I’m not exactly sure. It all happened so quickly.”
In a prototypical flow state, attention is focused outward and your conscious experience is associated with salient representations of the external objects of attention. They are disassociated from internally generated imagery. Michael Jordan was not daydreaming when he took that shot. When dreams, trauma or drugs induce dissociative states, it’s the opposite—we dissociate from representations of external objects rather than pay them attention. In both cases, thinking and metacognition are attenuated and the perception of time is altered—it’s common to exit from a flow state surprised at how much has elapsed.
We can think about these differences in terms of bottom-up vs. top-down information streams. Which kind of information stream dominates the neural tug-of-war determines the type of images that emerge in conscious awareness. For Michael Jordan, it was the bottom-up sensory stream entering his nervous system from the outside, giving him perceptions of the external world so clear and vivid that thinking was minimal. (This only worked because he was extremely well-practiced—the necessary motor programs were so ingrained they could be unleashed with fluid automaticity).
For someone in a drug, dream, or trauma-induced dissociative state, bottom-up streams are attenuated while top-down cognitive networks are disordered. The result is a failure to integrate sensory information about the external world into a coherent self-image, the thing that’s normally rendered during default waking experiences with metacognitive awareness.
There are shades of gray here. The contents of consciousness are not composed solely by bottom-up sensory inputs originating externally or top-down inputs generated internally. It’s always a mix. When the balance shifts sufficiently far in one direction, away from the typical balance associated with default consciousness, we recognize those experiences as non-ordinary states.
Phenomenology of Ketamine-Induced Dissociative States
Because ketamine is currently the object of intense study, we can use the latest neuroscientific research to help anchor us in the concrete world of biology.
Ketamine’s effects are dose-dependent, with a range of experiential effects more impressive than anything except psychedelics. At very low doses, rapid antidepressant effects are observed in both animals and people. A general dissociative state is not induced, but this does not mean dissociation is absent. Like the rubber arm illusion, this may be a form of simple dissociation, where one element of perception is dissociated from an otherwise normal waking state. Instead of physical sensation from your arm, what’s dissociated from your self-image is the negative emotional content characteristic of depression.
At higher but still relatively low doses—those commonly used in recreational settings—people report things like euphoria and light disorientation. At medium-ish but sub-anesthetic doses, dissociative states emerge. Sensory awareness of your surroundings is maintained, but with an odd feeling of disconnection. At the lower end of this range it may feel like your thoughts and reactions to external events are lagging (they probably are); at the higher end, more pronounced, out-of-body type experiences take place. Cognition is characterized by loose associations and unusual, tangential thought patterns, not unlike sleep-induced dream states.
When given to animals, medium-ish doses produce behavioral hallmarks of dissociation. In rodents, reflexes are unaffected, indicating intact sensory detection and motor capabilities, but emotional and motivational responses, like how quickly they remove an annoying piece of tape stuck to them, are diminished. They act as if they just don’t care much about their sensory inputs. This is probably the explanation for the analgesic (pain-reliving) effects of ketamine at these doses: The painful sensations are still there, just not associated with you and therefore not emotionally salient.
“People who are dissociating are aware of what’s happening. They’re not numb. They’re not unconscious. They’re not anesthetized. They perfectly register what’s happening to the body. They just don’t care because it’s not their self anymore. There’s a separation of stimulus detection from caring about it because it’s not attributed to the self.” -Karl Deisseroth, MD, PhD (M&M #35)
At high enough doses, anesthesia emerges. We tacitly assume people “knocked out” are devoid of conscious experience, but it’s impossible to prove. It may be that people’s capacity for multi-channel integration is so disrupted—they are so dissociated from sensory and emotional information streams—that metacognitive awareness is absent and the residues of experience fail to be encoded in memory. As anesthesiologist Dr. Alex Proekt pointed out, we can never be sure that the absence of behavioral responses to external stimuli—whether in deep sleep, anesthesia, or vegetative states—entails total loss of consciousness.
The phenomenological differences associated with distinct points along ketamine’s dose-response curve suggest that dissociation is not all-or-nothing. It can be so mild that one aspect of perception is dissociated from an otherwise sober, metacognitively aware state of mind; it can be moderate, with variable levels of behavioral or emotional detachment, but basic sensory perception largely intact; it can be so strong you’re in a truly altered state divorced from normal perceptions, difficult to describe later due to impoverished memory encoding. At some point along this continuum the pattern of waves in your mental pond has changed enough that we call it a dissociative state rather than a simple dissociation of this or that element of experience—differences that presumably correspond to changes in neural activity circumscribed to specific information streams vs. those involving larger swaths of the brain.
If dissociative states induced by medium range doses of ketamine preserve the ability to perceive external stimuli but disrupt the integration of sensory streams with higher-order cognitive networks involved in self-perception, how do patterns of neural activity in primary sensory areas—the middle of the neural stack—compare to those in association areas higher up? What kind of interference patterns does ketamine produce in the mental pond, and where?
Neuroscience of Ketamine-Induced Dissociative States
If you point a fancy microscope at an animal with a clear glass window surgically implanted into its cranium, you can watch what the brain does as it’s doing it:
Those are real brain cells (literally) lighting up in a pattern tied to the electrochemical flux governing whether they “speak.” The light comes from molecules engineered to emit photons whenever a neuron is sufficiently active. The light shoots out of the brain and back up through the microscope. Sensitive physical sensors record its intensity. Computer code analyzes each pixel of every recorded video frame, allowing the brain’s mysterious language to be quantified and decoded.
This type of thing is now routine. A number of labs have recorded what animals’ brains are doing when they receive dissociative, sub-anesthetic ketamine doses. When given to mice in states of quiet waking—when they’re just sitting there, not doing anything in particular—neurons across the cerebral cortex show an interesting switch. Here’s what the data looks like:
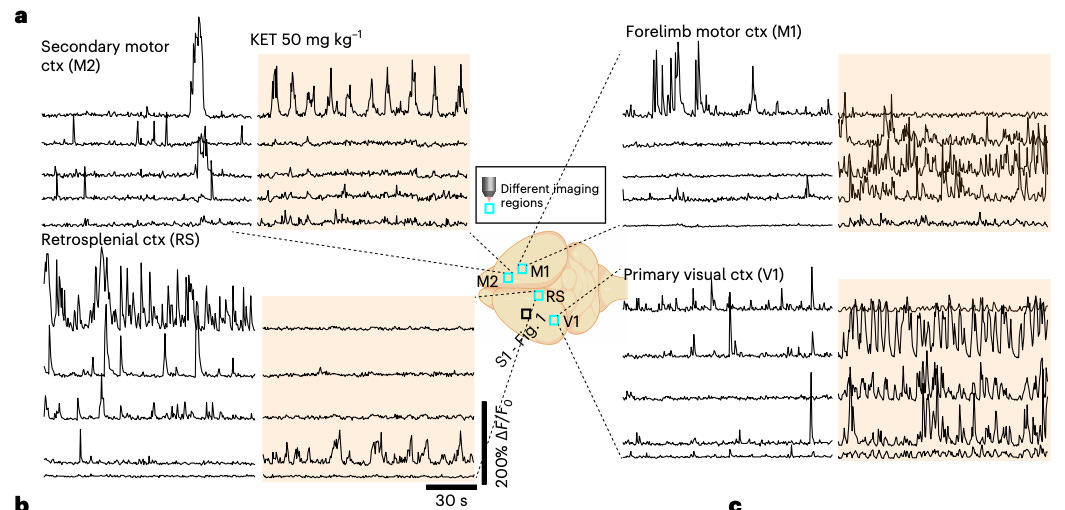
The black lines represent the activity of single neurons before (white background) and after ketamine dosing (shaded background). When they look like flat-ish squiggles, the neuron is largely inactive. When they show large upward deflections, the neuron is more active. Notice the basic trend: inactive neurons became louder in response to ketamine, while previously active neurons became quieter. This was true in sensory regions like primary visual cortex (V1), primary and secondary motor cortex (M1, M2), and a higher-order association area known as retrosplenial cortex (RS).
This shift was seen in excitatory neurons, cells that excite those they “speak” to, making them more likely to fire signals of their own. They make up the majority of our brain cells and their communication patterns are tightly controlled by smaller populations of inhibitory neurons. Ketamine affects both excitatory and inhibitory neurons such that excitatory neurons that were only lightly inhibited before tend to become more strongly inhibited, and vice versa.
A neuron’s activity is determined by how much electrochemical current flows through the large number of channel proteins embedded in its membrane. Different types of channels are specialized to allow certain combinations of ions (e.g. sodium, potassium, etc.) to pass through. By blocking specific channels, like NMDA receptors, ketamine alters the excitability of both excitatory and inhibitory neurons. End result: a change in the overall pattern of inhibition—previously quiet excitatory neurons become louder, previously loud excitatory neurons become quieter. (We’re simplifying, but that’s the gist).
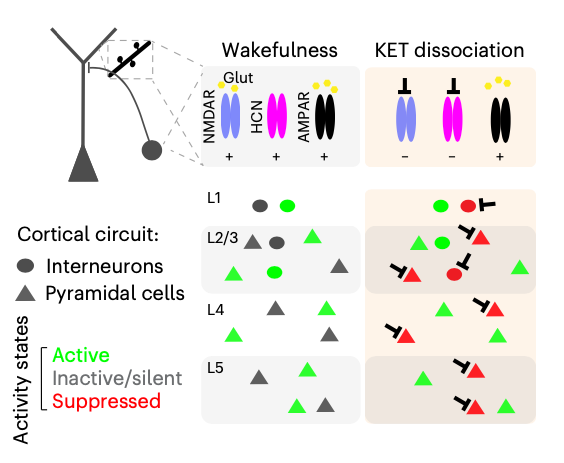
This ketamine-induced pattern is different from what is observed with hypnotic drugs. Such drugs increase inhibition generally, leading to a suppression of excitatory neurons across the board rather than a switch in whether neurons are active or silent. This switch in activity is seen with dissociation-inducing levels of ketamine—doses that are not anesthetic and do not induce behavioral signs of lethargy or the large, slow brain waves associated with non-REM sleep. The cerebral cortex is still “awake,” with large-scale activity patterns more closely resembling wakefulness or REM sleep than dreamless, non-REM sleep. If you double the ketamine dose, these patterns begin to look more like NREM sleep, with slower, large-amplitude oscillations.
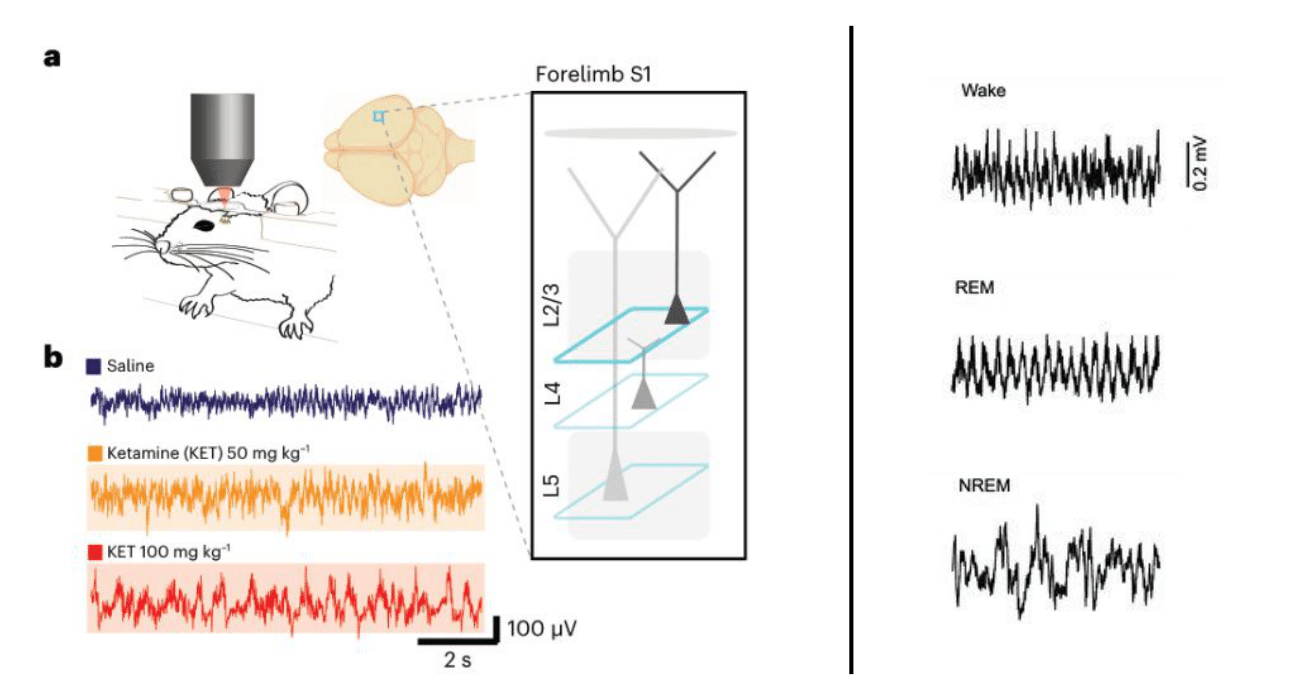
Are the mice also having a dissociative experience, with the loose associations and unusual patterns of cognition you would have on a comparable dose? The subjective experience of mice is forever inaccessible to us, but the data shows that ketamine induces measurable and reproducible changes to their brain state that track with behavioral hallmarks of dissociation. If mice can’t verbally report their subjective experience, how much insight into the nature of human psychological dissociation can this research really give us?
One way to get at this is to measure these things in mice and humans in parallel, looking for similarities. The question is twofold: is their a particular pattern of brain activity correlated with behavioral evidence of dissociation in mice and verbal reports of dissociative experience in humans? If so, can dissociative states be caused by injecting this pattern into specific parts of the brain?
Another research group conducted experiments aimed at these questions with the help of a medical patient suffering from epilepsy, whose seizures were often preceded by a dissociative state called an “aura.” The patient’s brain had previously been implanted with electrodes for diagnostic purposes, enabling scientists to study the waves of electrical activity that appeared during pre-seizure auras.
The patient’s own description of their subjective experience during pre-seizure auras:
I was listening to two parts of my brain speak to each other in a way that a third part of my brain, which I considered to be me, was able to listen.
What I considered me shrank to this other part of me where the other parts of my brain that were talking, I stopped considering them me.
During dissociative auras, scientists observed a particular rhythm of activity emerge in an area of the brain known as the deep posteromedial cortex, the human equivalent of mouse retrosplenial cortex. This rhythm was a regular, three-times-per-second (three hertz) oscillation, present when the patient reported being in a dissociative aura and only in this region. A similar oscillation was also seen in mouse retrosplenial cortex with sub-anesthetic doses of ketamine.
To move beyond correlation and demonstrate causation, scientists induced the same oscillation in the retrosplenial cortex, using electrical stimulation through the patient’s electrode implant and optogenetics in mice. In both cases, injecting this rhythm into retrosplenial cortex was sufficient to cause a dissociative state—mice showed behavioral hallmarks of dissociation, and the human patient said, “Yep, this feels like my auras.”
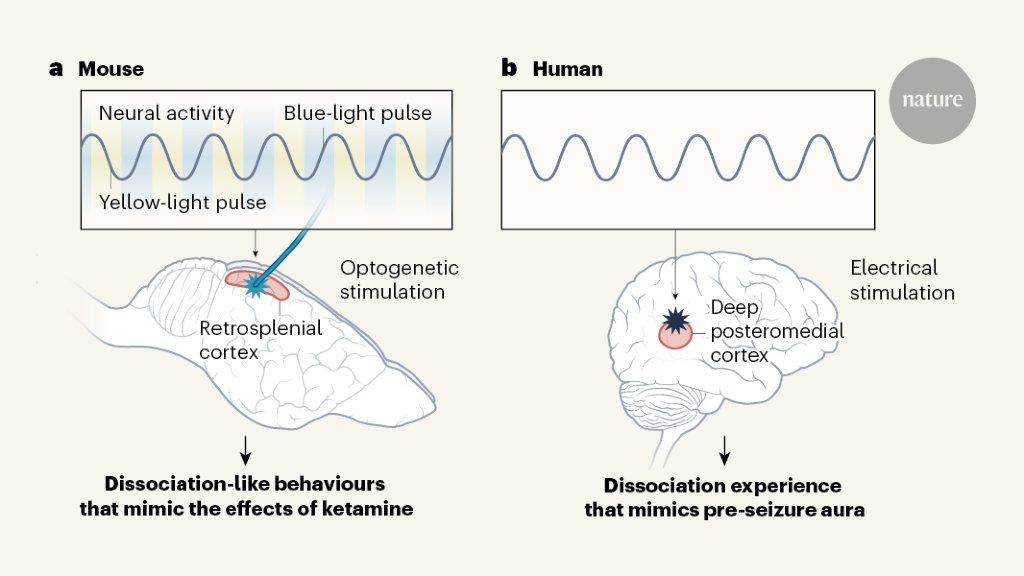
Together, these studies indicate that dissociation-inducing doses of ketamine cause a change in activity patterns throughout sensory, motor, and association regions of the cerebral cortex. In addition to this widespread population switch, a particular rhythm of activity emanates from neurons within one subsection of one region—the retrosplenial cortex (an association area).
Roughly speaking, different patches of cortex can be described as sensory, motor, or association areas. In relative terms, sensory areas specialize in processing information from one sensory modality, such as vision or touch; motor areas specialize in creating motor plans of action; association areas integrate information streams from multiple sensory and motor areas. Association areas probably do much of the processing of higher-order cognition and perception: generating a model of self, stitching disparate information streams into multimodal percepts, and coupling the appropriate emotional and cognitive responses to external objects constructed from pre-processed sensory data.
The observed neural patterns begin to paint a picture of how the hallmarks of dissociation can be explained in neurobiological terms. One hallmark is diminished responsiveness to external stimuli despite intact sensory and motor capabilities. External stimuli which normally elicit an emotional response, such as the avoidance of unpleasant sensations, fail to do so when animals are dissociated—emotional reactions are uncoupled from perceived sensations. This is consistent with the interpretation that the rhythm observed in retrosplenial cortex, sufficient to cause dissociation, represents a disruption of this multimodal association area’s ability to perform its normal, integrative function: coupling sensations to an adaptive emotional and behavioral output.
Another hallmark of dissociation is disordered cognition and unusual perceptions. In humans, this manifests as loose and tangential thought patterns, depersonalization & derealization, and hallucinations. In simplified terms, you can think of your perceptions as being some kind of higher-order pattern of activity in your cortex produced by the collision of multiple sensory and motor activity patterns in association areas. Whether induced by a drug, traumatic experience, or sleep-associated dream, dissociative states involve populations of neurons displaying activity patterns distinct from those seen during default wakefulness.
With ketamine-induced dissociation, animals are awake and behaviorally responsive (although in an unusual fashion). Their cortex is active, not asleep, with broad activity patterns more like those of waking or REM sleep than NREM or anesthesia.
The hallucinatory dream states of REM occur due to changes in the neurochemical environment of the brain, together with a general diminution of sensory streams flowing in from the outside world. Uncoupled from these information streams, different populations of neurons turn on and off in combinations not seen during sober wakefulness, giving rise to the weirdness of dreams: you experience fantastical images never perceived while awake and constellations of everyday percepts that don’t normally co-occur. Time is distorted. Images progress in strange, illogical sequences. There’s little metacognitive awareness. One moment your in bed, and then you’re building a go-kart with your ex-landlord.
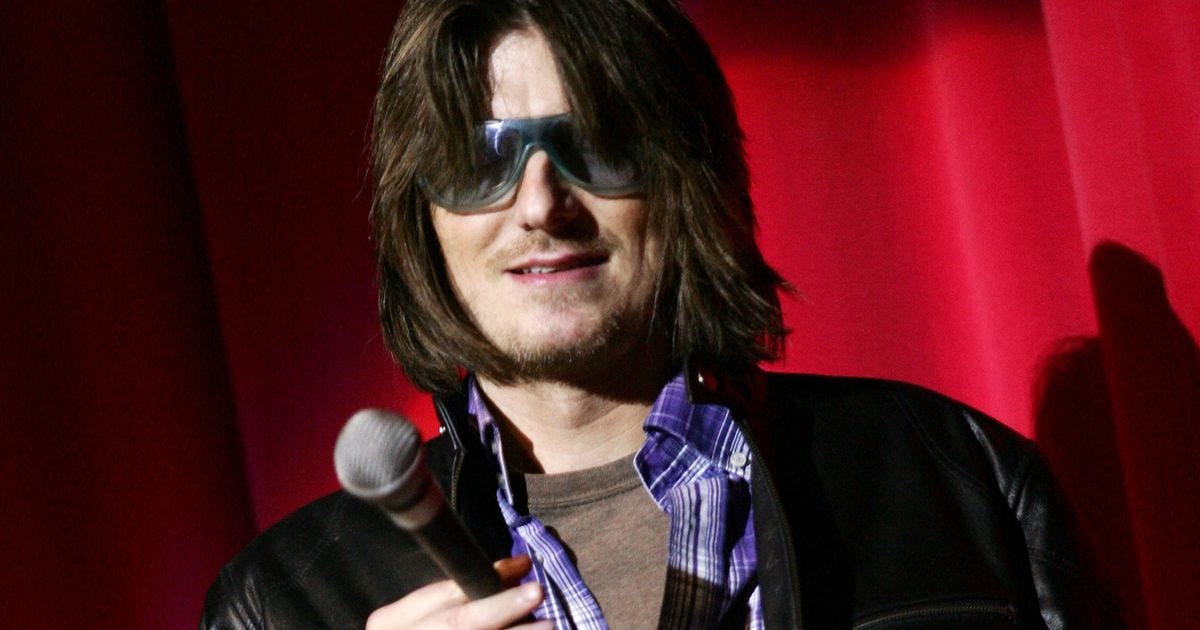
Like sleep-induced dream experiences, drug-induced dissociations involve an uncoupling of sensory information from normal emotional and cognitive processing. The difference is that this results from the pharmacological effects of the drug rather than diminishment of sensation altogether. Both states involve a pattern of constraints distinct from wakefulness—bottom-up sensory information streams make less of a splash in your mental pond. At lower dissociative doses, behavioral responsiveness is altered but external sensory inputs make a big enough splash to keep you mostly tethered to normal sensory perceptions. At higher dissociative doses (e.g. “k-holes”), perception and cognition become so untethered from sensory constraints that experience is perceptually divorced from your surroundings.
What is Psychological Dissociation?
Psychological dissociation is dreaming, awake.
Is it exactly like the dream states we experience during REM sleep? No. In a dissociative state, you’re not quite awake, but you’re not exactly in a sleep-induced dream state, either. It’s a transposition of modes of perception and cognition characteristic of dreams into the awake mind. Dissociation as a waking dream captures the psychological essence of what dissociative experiences feel like compared to states of consciousness we’re all familiar with.
Both sleep-induced dreams and dissociative states share these key commonalities:
Sensory information fails to be integrated into higher-order perceptual and cognitive networks as it does during normal wakefulness;
Cognition is disrupted such the brain’s ability to construct a self-image and narrativize experience in a way that facilitates memory encoding are impaired.
We can distinguish between different levels of dissociation based on their strength (how different they are compared to default consciousness) and the doses of dissociative drugs that induce them. These “levels” of dissociation blend into each other, but it’s convenient to think in terms of three broad buckets:
Simple (weak) dissociation: Specific elements of waking experience become dissociated from your self-image in an otherwise normal waking state, with intact cognition. Simple dissociations can be induced by low doses of a dissociative drug or by ‘hacking’ sensory perception.
Examples: Dissociation of physical sensations from your arm (rubber arm illusion) or dissociation from negative emotional appraisals of you self (low dose antidepressant effects of ketamine).
Dissociative states: General brain states in which your experiences become increasingly detached from information about the external world. Internal imagery more strongly dominates the contents of experience. Cognition and metacognitive awareness are disrupted to various degrees.
Examples: Psychological states induced by medium doses of dissociative drugs like ketamine, traumatic experiences, or certain neuropsychiatric conditions.
Strong dissociative states: Experience becomes completed detached from information about the external world despite the influx of information through sense organs. Cognition and metacognitive awareness are strongly disrupted or absent and you become dissociated even from your own memories and internal representations.
Examples: Psychological states induced by higher doses of dissociative drugs like ketamine (“k-holes”) or especially potent traumatic experiences.
Implicit in our exploration of dissociative states has been a way to think about personality and identity in behavioral terms, informed by neurobiology. An understanding of dissociation at the psychological and neurological levels provides us with a framework for understanding phenomena like Dissociative Identity Disorder. This will be the subject of the next essay.
To learn more about the topics covered in this essay, try these episodes of the Mind & Matter podcast:
Lisa Monteggia: Ketamine, SSRIs, Depression, Psychiatric Disorders & the Brain | #29
Christian Lüscher: How Does Ketamine Work & Is It Addictive? | #90
Alex Proekt: Consciousness, Anesthesia, Coma, Vegetative States, Sleep Pills (Ambien), Ketamine, AI & ChatGPT | #101
Karl Deisseroth: Psychiatry, Autism, Depression, Anxiety, Optogenetics, Dissociation & "Projections: A Story of Human Emotions" | #35
Read other installments of the ‘What is Psychological Identity?’ series:
Part II: Illusions, Phantoms & Dream Avatars
Part III: The Molecular Roots of Self-Identity
Part V: Coming soon